ِABS : Exploring the Hydrogen Value Chain and Hydrogen-based Fuels
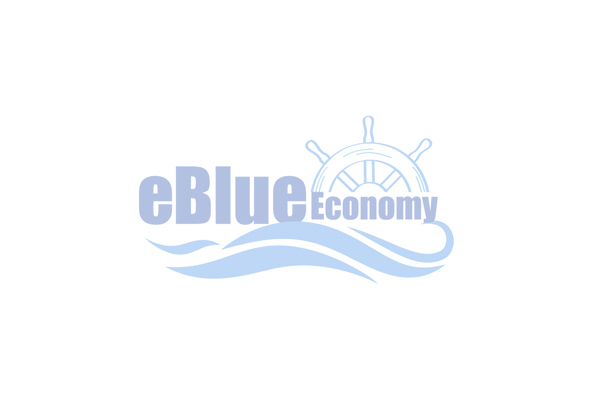
Hydrogen is expected to play a key role in meeting the International Maritime Organization’s emission reduction goals as well as play a significant part in the ongoing energy transition.
Not only can hydrogen be utilized in powering vessels but also in the production of other zero and low-emission fuels, such as ammonia and methane. Implementation of hydrogen as a marine fuel is still emerging and the infrastructure needed for it to meet the industry’s needs does not exist at scale yet
. A critical step to scaling up the hydrogen value chain is the development of regional hydrogen hubs. The location of these hubs must be carefully selected due to the energy and water inputs they would need to utilize. ABS reported
Hydrogen, which has traditionally been used as chemical feedstock in several industries, is now beginning to see wider use as an alternative fuel. It is well suited to produce electro-fuels (e-fuels).
A complete overview on hydrogen can be found in recent ABS publications, including: Hydrogen as Marine Fuel Sustainability Whitepaper — June 2021 and the newly released publication, Offshore Hydrogen Production of Green Hydrogen — February 2022.
Furthermore, the role of hydrogen as a marine fuel is expected to have a significant effect on the emerging energy transition. Developments related to hydrogen’s transportation, production, safety, standards and regulation are going to be in the spotlight for the years to come.
HYDROGEN PRODUCTION OVERVIEW
Hydrogen is produced through chemical reactions that separate it from water or hydrocarbons. In industry, it is often referred to by different colors to indicate its origins. The more common sources are:
• Brown hydrogen, produced via coal gasification or coal carbonization.
• Gray hydrogen, produced from reaction to the reformation of steam using natural gas.
• Blue hydrogen, produced in the same manner as gray hydrogen but the emissions are captured, resulting in a netzero carbon footprint from the reformation process.
• Green hydrogen, produced from renewable energy sources powering the water-electrolysis process with no carbon emissions.
• Pink hydrogen is generated through electrolysis powered by nuclear energy.
In 2019, global consumption of hydrogen fuel reached about 75 million metric tons (Mt) according to International Energy Agency (IEA). Of that volume, only 1.5 Mt was green hydrogen.
A market analysis performed in April 2021 by the Energy Transitions Commission (ETC), a global industry coalition committed to achieving net zero by 2050, indicated that the demand for hydrogen was expected to increase annually by seven to nine percent.
This would lead to an estimated demand between 500 and 800 Mt of hydrogen by the year 2050 and fulfill 15 to 20 percent of the global energy demand.
To reach a production level of 500 Mt by 2050, there would need to be 3,000 to 6,000 gigawatts (GW) of newly installed renewable energy sources devoted exclusively to hydrogen production.
EXPLORING THE HYDROGEN VALUE CHAIN AND HYDROGEN-BASED FUELS
Hydrogen, which has traditionally been used as chemical feedstock in several industries, is now beginning to see wider use as an alternative fuel. It is well suited to produce electro-fuels (e-fuels).
A complete overview on hydrogen can be found in recent ABS publications, including: Hydrogen as Marine Fuel Sustainability Whitepaper — June 2021 and the newly released publication, Offshore Hydrogen Production of Green Hydrogen — February 2022.
Furthermore, the role of hydrogen as a marine fuel is expected to have a significant effect on the emerging energy transition.
Developments related to hydrogen’s transportation, production, safety, standards and regulation are going to be in the spotlight for the years to come.
HYDROGEN PRODUCTION OVERVIEW
Hydrogen is produced through chemical reactions that separate it from water or hydrocarbons. In industry, it is often referred to by different colors to indicate its origins. The more common sources are:
• Brown hydrogen, produced via coal gasification or coal carbonization.
• Gray hydrogen, produced from reaction to the reformation of steam using natural gas.
• Blue hydrogen, produced in the same manner as gray hydrogen but the emissions are captured, resulting in a netzero carbon footprint from the reformation process.
• Green hydrogen, produced from renewable energy sources powering the water-electrolysis process with no carbon emissions.
• Pink hydrogen is generated through electrolysis powered by nuclear energy.
Different methods of hydrogen production.
In 2019, global consumption of hydrogen fuel reached about 75 million metric tons (Mt) according to International Energy Agency (IEA). Of that volume, only 1.5 Mt was green hydrogen.
A market analysis performed in April 2021 by the Energy Transitions Commission (ETC), a global industry coalition committed to achieving net zero by 2050, indicated that the demand for hydrogen was expected to increase annually by seven to nine percent.
This would lead to an estimated demand between 500 and 800 Mt of hydrogen by the year 2050 and fulfill 15 to 20 percent of the global energy demand.
To reach a production level of 500 Mt by 2050, there would need to be 3,000 to 6,000 gigawatts (GW) of newly installed renewable energy sources devoted exclusively to hydrogen production.
Focusing in particular on the maritime transport sector, the projection for hydrogen demand combined with the production capacity of the projects in the pipeline and forecast based on that, it seems that more capacity will be required to cover the hydrogen needs of the sector in the long term.
HYDROGEN PRODUCTION (GREEN/PINK) — ELECTROLYZERS
Hydrogen that is produced through the use of renewable electricity or electricity that is generated by nuclear power plants relies heavily on electrolysis. Electrolytic systems have been widely used for decades in the industry particularly in processes such electrowinning and electrorefining.
At the heart of the system, we find the electrolyzing unit which can use a range of technologies to produce electrolytic work.
Currently there are three commercially-viable designs of electrolyzers being considered for use in hydrogen production: proton exchange membrane (also known as polymer electrolyte membrane, or PEM) electrolyzers; alkaline electrolyzers (AEC); and solid oxide electrolyzers (SOEC).
Although PEM electrolysis is a mature technology and has a short response time for electrical load change, it is expected to remain relatively expensive because of excessive use of rare or costly metals (Ir, Pt, Ti). SOEC, though much less mature than AEC has significant potential.
It is anticipated to reach the same cost as AEC, while it will likely maintain an efficiency advantage
Each electrolyzer design has its own unique benefits and drawbacks. The selection of any particular design will influence the design of the complete facility and vice versa.
They each require different pre-processing techniques for the supplied water, have different operating conditions, and have different maintenance requirements.
In order to understand where each electrolyzer could be optimal, the complete hydrogen production facility must be examined.
As the building block of other energy carriers, hydrogen will add a significant cost element to the overall costs related to the final energy carriers and ultimately to the economics of the total energy conversion of the value chain.
Considering that the production of hydrogen will heavily depend on the electrolysis process, its costs will also be affected similarly.
Therefore, it is interesting to look at how electrolysis technologies can define the final cost of fuels produced.
For the purposes of this publication we look into proton exchange membrane (also known as polymer electrolyte membrane, or PEM) electrolyzers, alkaline electrolyzers (AEC), and solid oxide electrolyzers (SOEC).
The graphs below show the cost of H 2 production related to the levelized cost of electricity (LCOE) and the specific electrolysis technology.
We observe that due to the increased efficiency of SOEC, the cost of the final product is expected to be lower than AEC across a wide range of electricity prices. If we account for installation costs (capital expenditure [capex] incurred),
AEC performs better in 2020 but as the technology benefits from scale effects and installation cost drops, the expectation is that in 2050, SOEC will provide a more cost-effective solution.
The cost of transitioning to net zero is one of the biggest challenges for shipping companies. As technology continues to evolve and economies of scale are achieved, the cost of the alternative fuels will continue decreasing.
However, according to a recent study, the price of alternative fuels will remain more expensive than conventional marine fuels in 2050 .
GLOBAL GREEN AND BLUE HYDROGEN PROJECTS
Green hydrogen would provide a cleaner source of hydrogen feedstock and fuel for many industries, but realizing this prospect will require significant additional investment and infrastructure.
Projects to produce green hydrogen are on the rise and this trend is expected to continue for the years to come, as the production of gray hydrogen has already started to decline